PLA (Polylactic Acid)
Polylactic acid (PLA) is one of the most widely used and commercially successful bioplastics, as it exhibits good processability and mechanical properties. Compared to other biodegradable products, PLA has exceptional durability, mechanical strength, and transparency, making it one of the top choices among biodegradable materials. Furthermore, since lactides are formed from the microbial fermentation of agricultural byproducts (mainly carbohydrate-rich substances), PLA is considered a suitable alternative to petroleum-derived products.
What is PLA?
PLA is a derivative of lactic acid (LA), a linear aliphatic thermoplastic polyester. It is recognized and classified as one of the aliphatic polyesters, similar to polyglycolic acid (PGA), and is commonly manufactured from hydroxyl acids. Due to the asymmetric carbon atom in its molecule, LA exists in two forms, L-LA and D-LA. Additionally, through chemical conversion, PLA can be repolymerized when recycled back to lactic acid. Depending on the proportion and distribution of L- and D-LA in the polymer chains, the thermal and mechanical properties of PLA are justified. Due to methyl (-CH3) side groups in the polymers, PLA is considered hydrophobic in nature. Thus, the steric shielding effect of the methyl side groups makes it more resistant to hydrolysis compared to PGA. L-LA produces a semicrystalline polymer (PLLA), while poly(DL-lactide) (PDLLA) is an amorphous polymer.
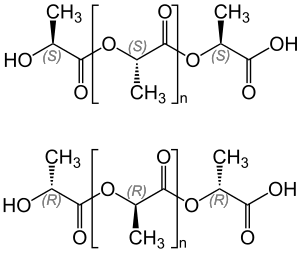
Properties
Since PLA is primarily composed of blocks of L-LA, the polymer is semicrystalline, and its structural regularity is high. At high temperatures, up to 37% of the crystalline regions provide increased mechanical strength. The glass transition temperature (Tg) is an important characteristic of polymeric materials, as it specifies the temperature above which the amorphous regions of PLA plastics become rubbery or soften, and cease to be brittle. The Tg of semicrystalline and amorphous PLA ranges between 50 and 70 °C. The half-life of degradation depends on the stereochemistry of PLA and the molecular weight, typically ranging between six months and two years. Additionally, as degradation time varies, mechanical properties also change. PLA is a transparent and rigid polymer with an elongation at break of 85% to 105% and tensile strength between 45 and 70 MPa. Regarding thermal properties, the melting point of PLA ranges between 170 and 180 °C, with a Tg of 53 °C.
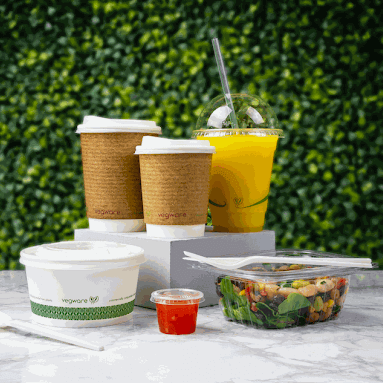
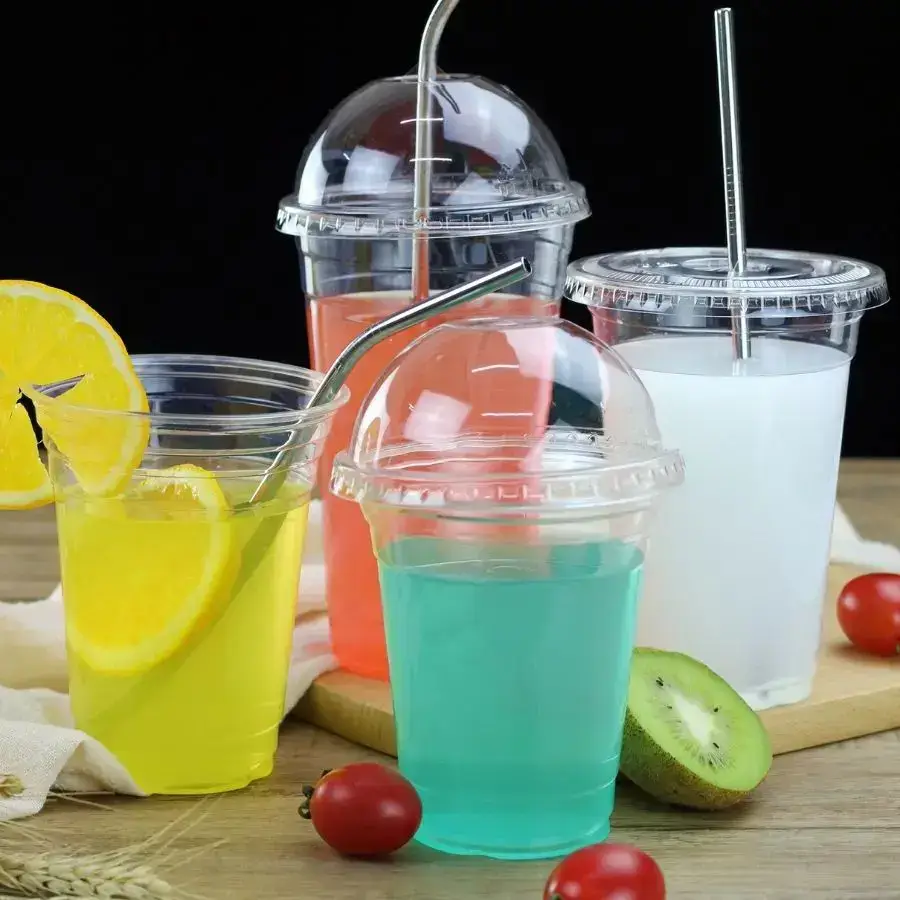
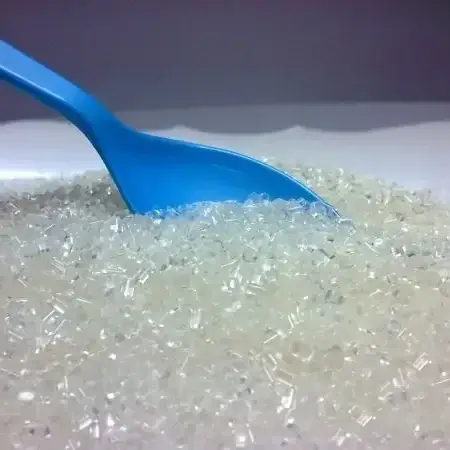
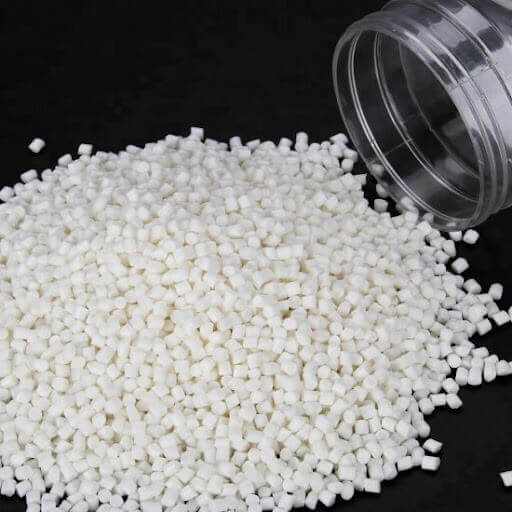
Processing Methods:
Regarding PLA processing methods, they are well-established polymer manufacturing techniques adopted from commercial polymer manufacturing techniques. However, various controls and applications must be followed to obtain all the benefits of the biopolymer. The most common methods used for manufacturing biopolymers are hot extrusion, injection molding, blow molding, and thermoforming.
Advantage over other aliphatic polyesters:
Compared to other aliphatic polyesters, PLA has demonstrated many excellent properties such as high mechanical strength and modulus, biodegradability, biocompatibility, and easy processing. The increasing application of PLA is also related to the improvement of its properties, such as heat resistance modification, copolymerization, and blend modification.
PLA in the Biomedical Industry:
The biomedical industry is among the sectors incorporating PLA bioplastic into their products. Several interesting properties have made PLA an ideal material for biomedical applications. One of them is its hydrolysis mechanism, by which PLA degrades naturally in situ. Thus, additional surgeries to remove the implanted device are not needed. Therefore, patient recovery rates can be improved, and healthcare system costs minimized. The natural biocompatibility of PLA helps reduce critical immune response acquisition. Since degradation products are formed by lactic acids and short oligomers, these familiar materials can be metabolized by the body. However, pure PLA may struggle to meet all necessary requirements in this field. Therefore, over the years, nanocomposites based on PLA as alternative materials have been widely researched. There are nanocomposites based on PLA with copolymers and nanocomposites as matrices. The enhancement of nanomaterials with their proper application, nanomedicine, is an emerging field of medical treatment today.
Also, an emerging area in human healthcare is tissue engineering, which deals with tissue and organ formation and regeneration. In tissue engineering, scaffolds play a crucial role as they act as supports for cell adhesion and growth. Blood vessels, bone regeneration, and the neural system are some of the biomedical branches that use scaffolds to assist in treatment. Due to its good biocompatibility, PLA materials are widely used as scaffolds. PDLLA and PLGA are among the most used biopolymers in tissue engineering due to their wide range of melting and glass transition temperatures. Additionally, aligned nanofibrous PLLA scaffolds have excellent potential in neural tissue engineering. In osteogenesis, PLLA has been exceptionally useful in promoting positive outcomes.
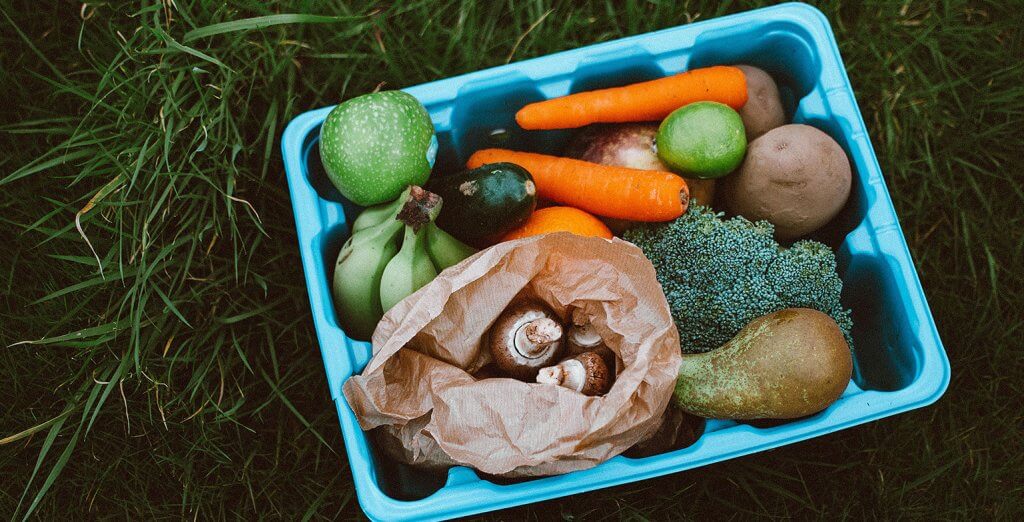
PLA in Packaging:
Another industrial application is in the field of packaging. Originating from lactic acid, PLA is a thermoplastic and biodegradable aliphatic polyester with great potential for packaging applications. The lactic acid monomer has two optical isomers, and the ratio between these two will determine PLA’s qualities as a packaging material. For example, high melting points and crystallinity will be obtained when using 100% L-LA monomers. Meanwhile, when using D/L copolymers at 90/10%, a polymerizable melt mass will be produced above its Tg, meeting bulk packaging conditions. As a packaging material, PLA is a good alternative and substitute for conventional plastics such as low-density polyethylene (LDPE), high-density polyethylene (HDPE), polystyrene (PS), and polyethylene terephthalate (PET). Good resistance to water solubility, high molecular weight, good processability, and biodegradability are attractive properties that make PLA an excellent packaging material. In terms of tensile strength modulus, flavor and odor barrier, PLA has properties similar to PE and PET or flexible PVC. Additionally, PLA has thermal stability and processability of PS, printability, and grease resistance of PE. Furthermore, for large-scale commercialization, PLA is one of the earliest biologically sourced polymers, and can be molded into objects, films, and injection-molded coatings. PLA can be recycled back to LA before undergoing repolymerization.
PLA in Agriculture:
PLA has also been used in the field of agriculture. The incorporation of plastic for agricultural applications is termed plasticulture and began in the 1950s to improve and increase production. There are several good reasons for the use of plastic in agriculture, including: 1) soil protection against erosion and plant protection against insects, birds, and weeds with mulch, 2) utilization of drip irrigation pipes, and 3) shielding of greenhouse tunnels. Previously, non-renewable traditional plastics were used as the main material and option in this industry. However, environmental effects have led biodegradable plastics such as PLA, PHA, and PBAT to become substitutes. However, pure PLA is not substantial for this industry, as the poor mechanical and thermal properties of homopolymer PLA have limited its use in the plasticulture industry. Thus, commercial mulch films based on PLA are manufactured by blending PLA with other biodegradable polyesters. Plasticizers are typically used to manufacture commercial mulch films based on PLA, and they are biodegradable as LA derivatives or oligomers have formed. However, plasticulture remains a developing field. Many aspects need improvement, such as the high cost of polymers. The use of bioplastics in agriculture is relatively new, and its implementation has not yet matured.
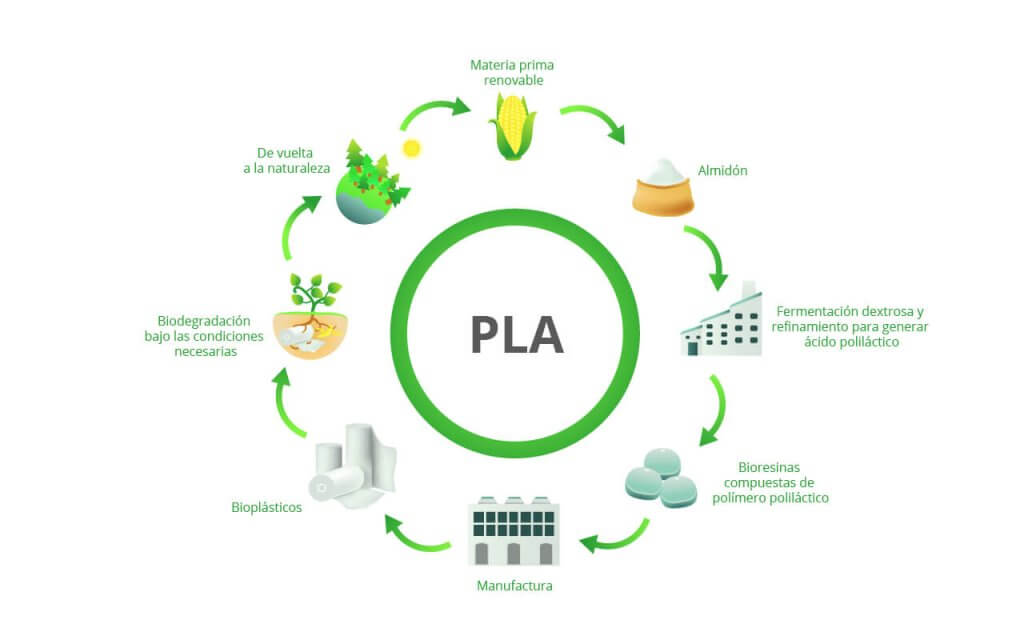
PLA in the Automotive Industry:
Furthermore, the automotive industry has also shown interest over the years in including materials of natural origin in its parts. These materials demonstrate excellent performance and contribute to improving fuel efficiency, as vehicle weight can be reduced. This also helps to decrease greenhouse gas emissions into the atmosphere. Some of the bioplastics available in the market are suitable for use in the automotive sector, such as PLLA and its compounds. Its excellent biodegradability/recyclability and attractive mechanical and physical properties are some of the key points that make PLLA an excellent choice for this industry. However, PLA still lacks in terms of fragility and thermal stability. Thus, by using suitable modifiers and additives, these issues can be overcome. Many automobile manufacturers like Ford, Mazda, Toyota, and Hyundai Motors have incorporated bio-based PLLA blends to manufacture various components. PLLA could also be used for interior car parts. By utilizing PLA, BIOFRONT could also manufacture automobile components such as doors, tread plates, and dashboards.
PLA, an Eco-Friendly Material:
The main advantage of using PLA is its respect for its eco-friendly nature. As it is derived from renewable resources such as corn, wheat, or rice, it can be used without worrying about depletion. Additionally, it is also biodegradable, recyclable, and compostable, indicating that there is almost no environmental impact during its disposal. The next advantage relates to the biocompatibility properties of PLA. This property is especially advantageous for biomedical applications. The material has no toxic or carcinogenic effects on the human body. When PLA degrades, it breaks down into H2O and CO2, without interfering with tissue healing. However, despite being considered one of the best materials to be used in industries, PLA has some drawbacks.
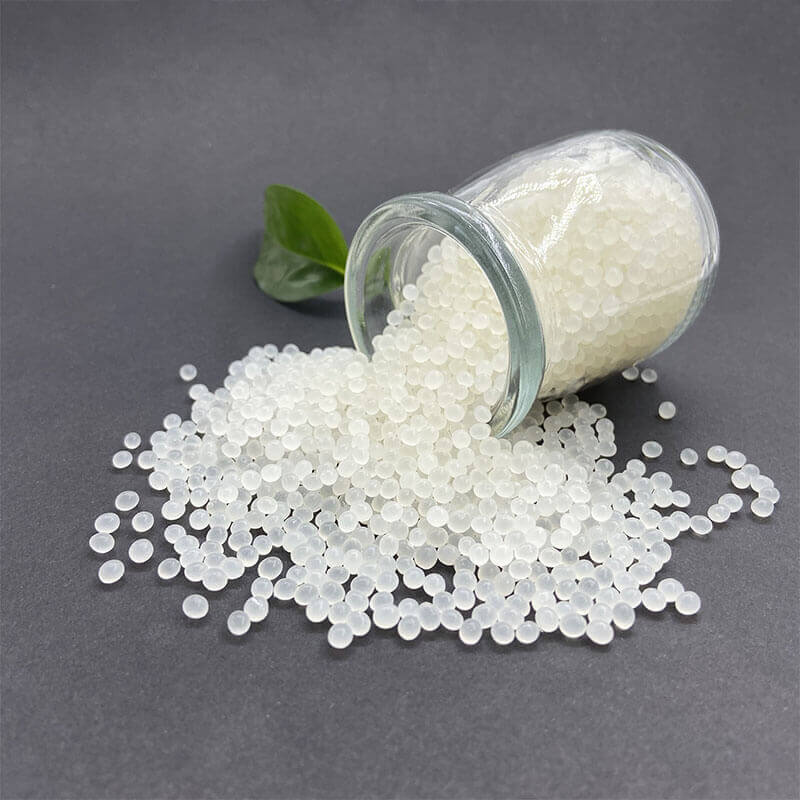
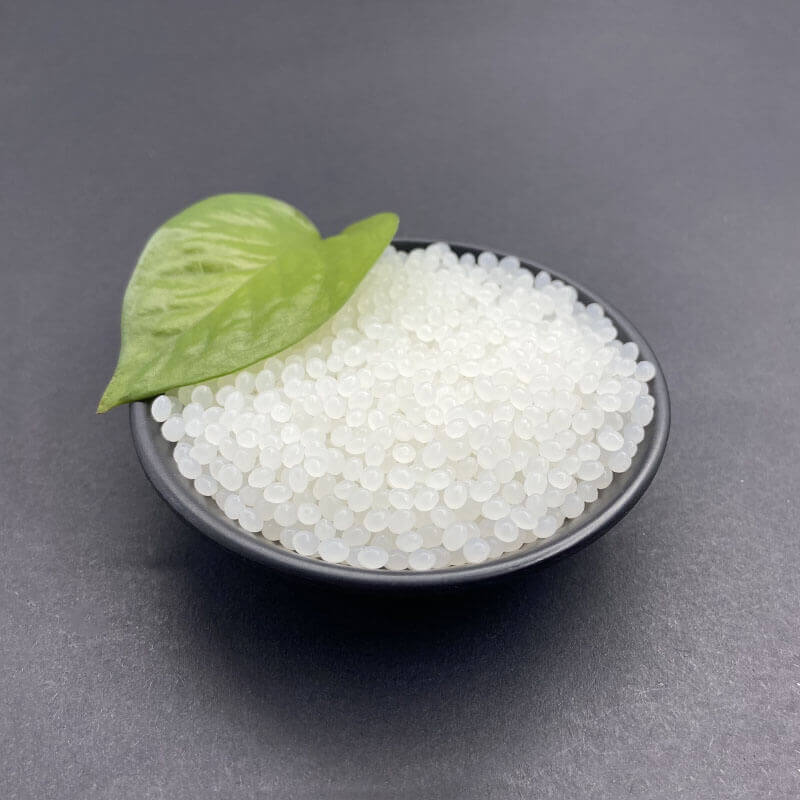
Drawbacks of PLA:
Firstly, it breaks with an elongation of less than 10%, demonstrating that it is very brittle and limits its use in various applications. Therefore, PLA has limited applications for products that require plastic deformation at higher stress levels, such as screws and fracture fixation plates in the biomedical field. Secondly, PLA also has a low degradation rate. The degradation rate of PLA is affected by its crystallinity, molecular weight and distribution, morphological properties, water diffusion rate in polymers, and stereoisomeric applications. PLA degrades through hydrolysis of the main chain ester groups, and the degradation rate is prolonged, with a lifespan of 3 to 5 years. This low degradation rate is unusual in the biomedical field and can lead to an inflammatory tissue response in living hosts due to its strong hydrophobicity, with a static water contact angle of approximately 80°, and direct contact with biological fluids.
PLA Production Worldwide:
Currently, NatureWorks, based in the USA, operates the world’s first industrial-scale PLA production plant. It was established in 2002 with a PLA line capacity of 70,000 metric tons. In 2015, its capacity was expanded to 150,000 tons. The PLA plant in Thailand, a joint venture between Total and Corbion, is the second largest, with a production capacity of 75,000 tons of PLA. Meanwhile, in China, a 5,000-ton PLA line was established by Hisun and added a 10,000-ton line in 2017. Additionally, in 2018, several lactide to PLA fiber lines were built by Hengtian with a capacity of 10,000 tons, while COFCO installed a lactide to PLA plant with a total of 10,000 tons in the same year in China. Furthermore, Synbra has launched a 5,000-ton line for the production of expandable PLA (BioFoam TM). It is projected that by 2025, the global biodegradable plastic market will reach $6.73 billion from $3.02 billion in 2018, more than doubling. The growing demand for biodegradable polymers in emerging economies such as Brazil, China, and India is the main driver of this spectacular increase. PHA and PLA are said to be the main contributors to the expansion of bio-based biodegradable plastics. The market shares of PHA and PLA are 1.2% and 13.9%, respectively. Both materials are expected to experience an increase in global production, with PLA production increasing from 293,290 tons in 2019 to 317,000 tons in 2024, equivalent to an 8% increase in production.
Sustainable Rejuvenation: The Impact of PLLA in Modern Aesthetic
Poly-L-lactic acid (PLLA) has emerged as a revolutionary component in the field of aesthetic medicine and reconstructive surgery, offering an advanced solution for facial and body rejuvenation without the complications of surgery. This biodegradable polymer acts as a powerful bio-stimulator, inducing the production of collagen type I and II, which are essential for maintaining skin structure and elasticity. Unlike traditional fillers that provide temporary and superficial results, PLLA works from within, improving skin quality in the long term.
Its mechanism of action begins with a controlled inflammatory response triggered by material injection. This response is crucial for the bio-stimulation process, as it activates fibroblasts to produce new, high-quality collagen. As PLLA is gradually reabsorbed, it leaves behind a robust collagen framework that supports the skin, resulting in a younger and firmer appearance.
In addition to its use in improving skin texture and reducing fine lines and wrinkles, PLLA has proven effective in treating body areas. Its application in the buttocks, arms, thighs, and other areas allows not only for improving skin texture and tone but also for reshaping and contouring the body, offering a non-surgical alternative for those seeking aesthetic enhancement.
The success of PLLA lies in its ability to provide natural and long-lasting results, making it an attractive option for those wishing to rejuvenate their appearance without resorting to surgery. Its safety, effectiveness, and the added benefit of being a biodegradable product compatible with the human body underscore its position as one of the most advanced and environmentally friendly options in the field of aesthetics.
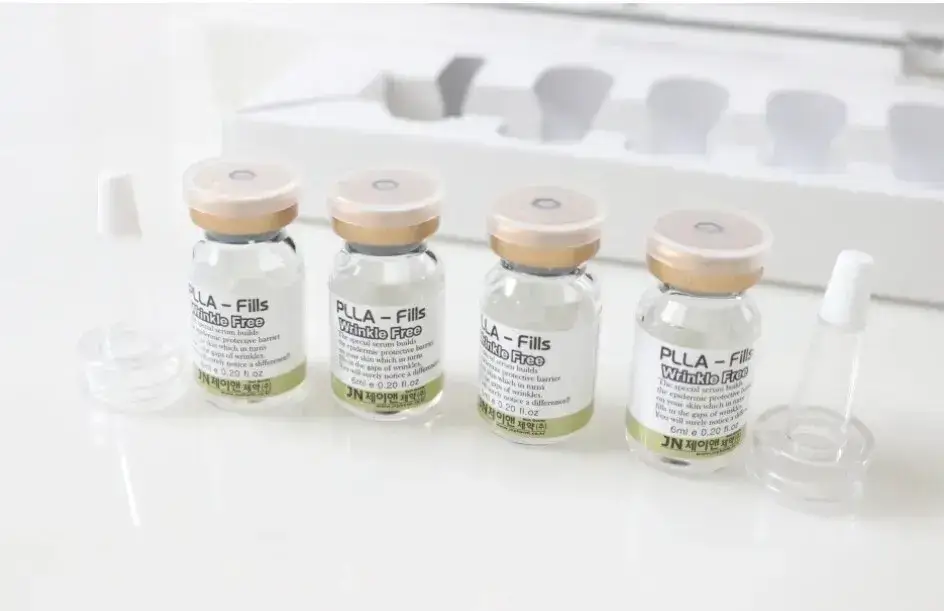